From Theory to Cosmos: How MRR Unlocks the Chemistry of Space
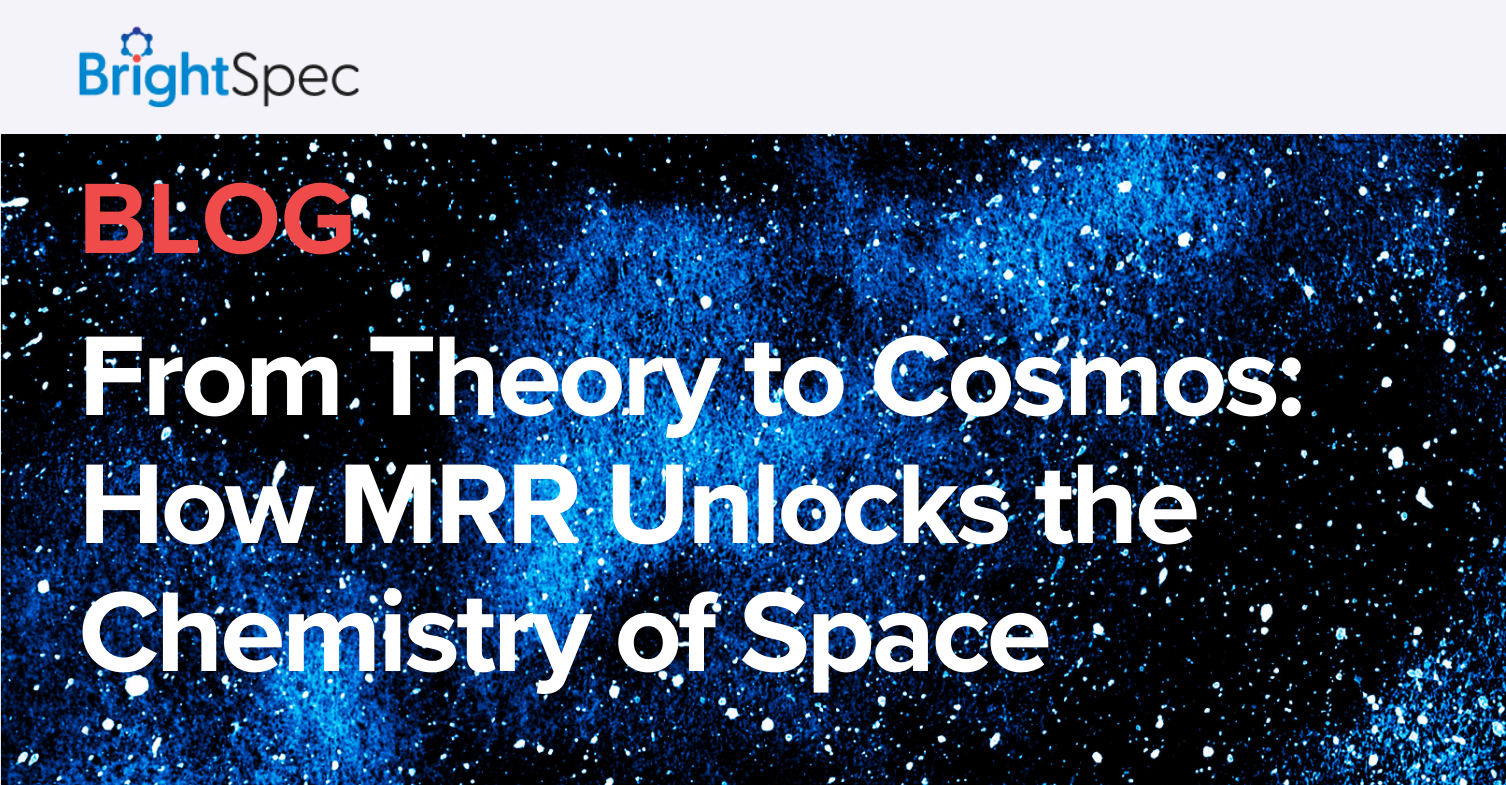
Astrochemistry—the study of chemical interactions in space—dives deep into questions about the universe’s origins and the processes that might have sparked life. Scientists study molecules in space to understand how these interactions could have seeded life on Earth and to explore the unique chemistry going on in different cosmic environments.
Studying molecules that are light-years away comes with major challenges. This is where rotational spectroscopy—and more recently, Molecular Rotational Resonance (“MRR”)—comes in as a game-changing tool.
Today, leading astrochemists use rotational spectroscopy to answer profound questions, including one of the biggest: How did life form on Earth?
A New Era for Astrochemistry
Astrochemistry took off in the 1960s when scientists discovered that complex, organic molecules existed in interstellar clouds. By the early 1970s, rotational spectroscopy became crucial for identifying these molecules based on their unique rotational “fingerprints.” As of now, over 300 molecules have been identified in space, most by rotational spectroscopy (you can see the full list here.
In the 2010s, a team of scientists specializing in rotational spectroscopy at the University of Virginia saw an opportunity to make this technology more accessible and founded BrightSpec. BrightSpec specializes in a specific form of rotational spectroscopy called Molecular Rotational Resonance (MRR), which has since found applications across industries, including pharmaceuticals, consumer goods, and academia. Today, for the first time, MRR is also a central method in published astrochemistry research.
Learn about how MRR technology works -->
Why Rotational Spectroscopy for Astrochemistry?
In astrochemistry, where the most complex and intriguing chemistry takes place in cold, dark clouds dense with dust, rotational spectroscopy becomes essential. Unlike infrared (IR) or electronic spectroscopy, which require light to penetrate, rotational spectroscopy can reveal molecular structures in these obscured regions where visible, IR, and UV light cannot reach. Molecular Rotational Resonance (MRR) stands out due to its unmatched specificity, enabling astrochemists to precisely identify the composition of specific cosmic regions based on unique spectral fingerprints.
MRR’s strengths are especially critical as astrochemists do not rely solely on theoretical calculations to identify new compounds in space. Instead, they first measure these compounds' spectra in the lab, using advanced instruments to recreate the exact spectral signatures of molecules that might exist only fleetingly under Earth-like conditions. This lab work is vital because the frequencies detected from space can shift due to the Doppler effect, as the molecular clouds move relative to Earth. To address this, astrochemists use lab-generated MRR spectra of unstable molecules for comparison, helping to detect and catalog these compounds in space with extraordinary accuracy.
Detecting Carbon in Distant Interstellar Clouds
Polycyclic aromatic hydrocarbons (PAHs) present astrochemists with a mystery and a promising avenue in understanding carbon sources in space. Studies like a recent one led by Prof. Brett McGuire at MIT are beginning to unravel the role of PAHs in the cosmos. McGuire’s team successfully detected 1-cyanopyrene, a large PAH, in the interstellar cloud TMC-1, which closely resembles the primordial cloud from which our solar system may have formed. This discovery is particularly intriguing as PAHs have long been theorized as a primary reservoir of carbon, a foundational element in organic chemistry and potentially life.
The detection of pyrene in both TMC-1 and the asteroid Ryugu supports the idea that PAHs may be widespread carbon sources, potentially seeded from interstellar clouds to rocky bodies in forming solar systems. Pyrene is estimated to account for about 0.1% of all carbon in TMC-1.
This research also connects to the detection of fullerene molecules like C60 and C70 (buckminsterfullerene), well-known in chemistry for their unique structure and stability. These fullerenes, previously identified in interstellar environments, add to the growing evidence of diverse carbon forms in space. Their detection, alongside PAHs, emphasizes the richness of carbon chemistry beyond Earth and brings us closer to understanding how these molecules might contribute to the formation of life-supporting systems.
McGuire’s team used the Green Bank Telescope (GBT) to analyze molecules in TMC-1 via their rotational spectra. Because pyrene’s symmetry makes it undetectable by standard radio astronomy, the researchers turned to a slightly modified version of it, cyanopyrene, which has a broken symmetry that allows it to emit detectable rotational signals. They first synthesized cyanopyrene in the lab, creating a precise spectral "fingerprint" to search for in space. This discovery not only marks pyrene as the third-largest molecule identified in space but also highlights rotational spectroscopy's capacity for detecting complex organic molecules across vast distances and under extreme conditions.
nanoMRR and the Discovery of a Missing Molecular Conformer
While McGuire’s work relied on rotational spectroscopy, other astrochemists are leveraging BrightSpec’s Molecular Rotational Resonance (MRR) technology to unlock their own chemical insights. Recently, a study led by Chris Medcraft became the first published astrochemistry research using BrightSpec’s nanoMRR instrument. The team examined vinyloxirane (VO), a molecule similar to propylene oxide—the first chiral molecule detected in space. Astrochemists are particularly interested in VO as a promising candidate for identifying a second chiral molecule in space. Chiral molecules, which exist in “left-” and “right-handed” forms, are crucial in the study of life’s origins because chirality is a characteristic shared by many biomolecules, such as amino acids.
To analyze VO’s structure, Medcraft’s team used MRR to examine its microwave spectrum within the 18-26 GHz frequency range. The analysis led to several discoveries, including the detection of a previously unknown conformer, called gauche-1, as well as a more detailed analysis of the anti conformer, which was already known. VO was found to have three main conformers: anti, gauche-1, and gauche-2, although only the first two were detected in this study. The anti conformer emerged as the most stable form, while the newly identified gauche-1 conformer is slightly less stable but exhibits a unique shape that enables internal stabilization.
Using MRR, Medcraft’s team assigned and fit rotational transitions for the gauche-1 conformer, expanded the transition range for the anti conformer, and identified vibrational satellite transitions of both conformers. The ability to detect and analyze these different molecular forms without needing to collect physical samples from the cosmos highlights MRR’s value in expanding our understanding of complex molecules like VO in space. This research could be key to eventually detecting VO in interstellar space and enhancing our knowledge of chiral molecules across the cosmos.
MRR: Bridging Theory and Reality in the Classroom
For chemistry educators, MRR provides a unique opportunity to demonstrate the connection between theory and reality. By helping students identify molecules through their rotational spectra, MRR turns abstract concepts of molecular structure into observable data. This is especially valuable in astrochemistry, where students can see how MRR helps scientists detect molecules remotely, based on spectral “fingerprints,” even without physical samples.
Astrochemistry offers an inspiring context for MRR as students explore complex topics, like chirality and symmetry. With MRR, students can observe how molecular properties translate into measurable spectra, illustrating the power of rotational data to reveal molecular structures in space. For professors, teaching with MRR brings these concepts to life, giving students a hands-on experience of how scientists use rotational spectra to identify and study molecules across vast distances.
Read the Q&A with Associate Professor Twagirayezu -->
Inspiring Future Chemists with Astrochemistry and MRR
Astrochemistry gives students an engaging, real-world application for rotational spectroscopy. By bringing MRR into the classroom, professors can help students tackle complex questions, like how molecules might have seeded life on Earth or contributed to the formation of our solar system. MRR’s ability to analyze interstellar molecules gives students a unique perspective on how chemistry can answer some of the universe’s biggest questions.
Adding another layer of insight, MRR’s precision allows for analysis of isotope ratios within these molecules, providing essential information to trace cosmic material. Isotope ratios, such as those for carbon and hydrogen, serve as tracers that offer clues about a molecule’s origin and the environmental conditions it has encountered. This method is especially relevant in astronomy, where isotope ratios can reveal the history of interstellar clouds or the movement of molecules across cosmic distances.
With BrightSpec’s MRR instruments, professors can offer students hands-on experience with cutting-edge technology. This not only enhances their understanding of molecular structure but also sparks curiosity about the future possibilities of science—from the lab to the far reaches of space.
Get in touch with the BrightSpec team -->